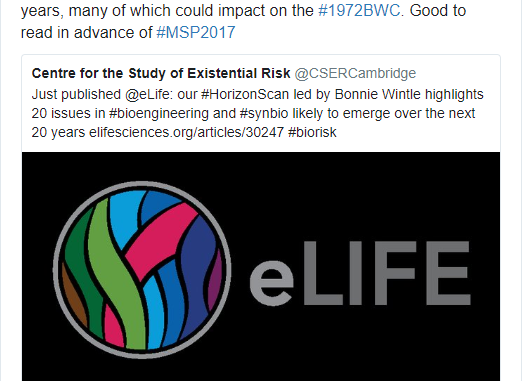
Wintle, B.C. & Boehm, C.R., Rhodes, C., Molloy, J.C., Millett, P., Adam, L., et al.., Sutherland, W.J. (2017) A transatlantic perspective on 20 emerging issues in biological engineering. eLife.
https://elifesciences.org/articles/30247
Issues related to information security:
Rise of automated tools for biological design, test and optimisation
The process of designing, testing and optimizing biological systems needs to become more efficient. Automation has been applied with great success to the design, test and manufacturing processes used in, for example, the automotive, aerospace and electronics industries. However, the engineering of organisms is not yet performed at similar scales. Automated fabrication with biological materials and the subsequent characterization of engineered materials and cells is now establishing itself in the form of services provided by ‘biofactories’ in a number of universities and companies (Check Hayden, 2014). Currently, the majority of laboratory automation is based around the use of existing tools and technologies, automating previously manually-executed protocols for design, simulation, building and testing. The next wave of lab automation will extend this, shifting experts’ focus from the minutiae of organism design and construction to a more abstract functional view; artificial intelligence-based software will automatically design and analyse experiments; and lab work will be performed by technicians or robots as instructed by the software. Hence, these tools make it possible to interrogate increasingly large experimental spaces rapidly and cheaply. This underlying technology will speed up the process to discover new molecules or prototype new applications fostering the development of many bio-based products. It will reduce the specialist skills needed for design, fabrication and validation and, along with outsourced fabrication, open up opportunities for countries with lesser biotechnology capabilities to take advantage of the booming bioeconomy. More broadly, the imminent arrival of “design for manufacturing” for bioengineered systems is likely to rapidly improve the ability of biomanufacturing to compete against traditional manufacturing industries (Carlson, 2016; Sadowski et al., 2016). The resulting acceleration of bioengineering will also impact the regulatory system as the complexity and rate of submissions rises.
Biology as an information science: Impacts on global governance
The ability to chemically synthesise or ‘write’ DNA molecules at low cost means the inherent value of any given DNA sequence lies increasingly in information about its function or the function of any product it encodes rather than in a physical sample of the organism from which that sequence originated. Genetic information can now be accessed online and exploited in a remote location without engaging with complicated export/import procedures or material transfer agreements. While the use (and misuse) of genetic information historically required the transportation of specimens, today’s biological engineers increasingly order the de novo synthesis of any DNA sequences that they wish to use from a commercial provider, using the sequence resources held in online databases as the template. Moreover, it is now possible to travel with a hand-held sequencer and to go from sample to sequence in less than 24 hours (Quick et al., 2016) negating the need to transport samples back to the laboratory to obtain the necessary genetic information. The enormous benefits of this rapid online transmission and synthesis of genetic information are already being realized, for example, through the production of ten million doses of vaccine just a month after receipt of an email containing the sequence of the viral strain (Powell, 2015). However, current practices and guidelines for governing access, privacy and benefit sharing from the use of genetic resources, such as the Nagoya Protocol (see below), are still predominantly focused on physical samples, increasing the potential for biopiracy.
Intersection of information security and bio-automation
Biological engineering puts genetic information at the heart of an iterative design-build-test cycle for genetically modified organisms. Advancements in automation technology combined with faster and more reliable engineering techniques have resulted in the emergence of robotic ‘cloud labs’ where digital information is transformed into DNA then expressed in some target organisms with very high-throughput and decreasing human oversight. This increased reliance on bio-automation and ingestion of digital information from multiple sources opens the possibility of new kinds of information security threats. These could include: tampering with digital DNA sequences leading to production of harmful organisms by researchers who are unaware of the malicious changes; sabotaging vaccine and drug production through attacks on critical DNA sequence databases or equipment; using DNA as a ‘Trojan horse’ to carry out a digital attack. The latter scenario was recently simulated by researchers from the University of Washington, who successfully engineered a DNA sequence to exploit a vulnerability they introduced into DNA sequencing software (Ney, 2017).
Information security is arguably a well-recognised threat so one might question why it is a horizon scanning issue. Emerging digital DNA tools and services present clear potential for new forms and sources of risk as DNA is directly ‘executable’ and verification methods such as sequencing can themselves be hacked, hampering efforts to assure quality and consistency. Recent experiences with ‘internet-of-things devices’ suggest that security does not always receive sufficient attention when a new technology is undergoing rapid development and increased decentralisation (Department of Homeland Security, 2016). Since bio-automation is currently undergoing such development and decentralisation, we propose that information security qualifies as an issue and that routes to tackling it should be explored as a priority. These might include setting information security standards for the bioindustry, such as ensuring strong encryption and quality control for all bio-automation, recognizing public bioinformatics databases as critical infrastructure, and further engagement with information security experts when implementing tools and services.
Effects of the Nagoya protocol on biological engineering
The Nagoya Protocol on Access to Genetic Resources and the Fair and Equitable Sharing of Benefits Arising from their Utilization (Convention on Biological Diversity, 2010), a supplementary agreement to the Convention on Biological Diversity (CBD), entered into force in 2014. It is expected to change the way genetic materials are treated by countries that are signatory to the protocol (93 at the time of writing). While many countries are still formulating national legislation and implementation plans, some countries rich in biodiversity (so-called provider countries) have already taken legislative steps to restrict access to physical and digital genetic resources originating from within their borders (Bagley and Rai, 2013; Manheim, 2016). Should the Nagoya Protocol be extended to associated data (such as genetic sequence information), it will substantially affect the collection, handling and transfer of such data which is used extensively in biological engineering. ‘Digital Sequence Information of Genetic Resources’ was discussed at a CBD meeting in December 2016 (the COP13 meeting) and recognised to be a cross-cutting issue (Convention on Biological Diversity, 2016). The decision was made to establish an Ad Hoc Technical Expert Group to compile relevant views and information, and a fact-finding and scoping study will likely be considered at the COP14 meeting. Regulatory uncertainty, restrictive terms set by provider countries, and limited capacity to deal with requests may slow down future research and its commercialization. In response, new programmes coordinating exchange of genetic resources may be implemented, potentially requiring an international system for tracking the origin of a genetic resource. Amidst its practical challenges, the developments discussed in ‘Biology as an information science’ (above) underscore the importance of maintaining the spirit of the Nagoya Protocol, for its potential to reduce inequality among countries and promote ecological sustainability by creating an incentive to preserve potentially valuable sources of genetic material.
Corporate espionage and biocrime
Cutting-edge biotechnology is associated with a range of concerns about criminal misuse. Emergent bioeconomies will face many of the same hazards and vulnerabilities as more established sectors due to the high cost of biotechnology product development. The comparatively demanding regulatory environment in areas such as food and health may make the field particularly susceptible to both corporate espionage and the emergence of counterfeit markets. In a recent example, one of six Chinese nationals charged by the US Government pleaded guilty for attempting to steal trade secrets from GM seed companies (Waltz, 2016). Beyond theft of physical samples, the information-centric character of modern biotechnology entails increased risk of cybercrimes such as data theft and extortion (Evans and Selgelid, 2015). Underground trading already exists for recreational drugs, medicines, and crop seeds (Tatge, 2004). With continuing expansion of the biotech industry and increasing accessibility of both biological information and genetic engineering techniques to non-specialists, bio-piracy will likely become more widespread in the future.
Challenges to taxonomy-based descriptions and management of biological risk
Today, efforts to describe and manage biorisk are based upon taxonomic classification of the agents involved (for example, the Australia Group’s ‘Lists of pathogens’ or the CDC’s ‘Select Agent Rules’). As the life sciences advance, the utility of these lists is diminished due to several factors. To begin with, chimeric and modified agents do not fit easily into such lists. For example, a virus composed of genetic elements from several related strains defies taxonomic description (e.g. is it a strain of measles virus, rinderpest virus or canine distemper virus?) and challenges safe handling guidelines. Secondly, and perhaps most fundamentally, it is the presence of particular functional properties of an agent that drive the risk, rather than the identity of the agent itself. For example, most strains of Bacillus cereus are harmless, but the identification of toxigenic strains (Okinaka et al., 2006) has prompted the US Centers for Disease Control and Prevention (CDC) to rewrite rules and include this strain as a select agent (US Department of Health and Human Services, 2016). Likewise, they have had to include at least one phenotypic definition, for Newcastle Disease Virus. The feasibility of describing and managing biorisk according to biological function, rather than taxonomy, has been the subject of a long-running debate (National Academies, 2010), but is extremely relevant at a time when many new pathogens and strains are being discovered through bioprospecting, and the current system risks overregulating harmless non-pathogenic organisms or failing to capture distantly related pathogens with similar properties. To ensure that biorisk management and biosecurity regimes remain relevant into the future, taxonomic lists would benefit from supplementary phenotypic definitions that capture the traits that influence the strains’ biosafety or biosecurity risk.
Shifting ownership models in biotechnology
Models of ownership in bioengineering are typically strongly vertically integrated and rely heavily on the patenting of both tools and applications. The current market structure and supply chain provides little access to basic bioengineering tools and technologies to those in low-resource settings who could arguably reap the greatest social and economic benefit from developing a sustainable bioeconomy based on local needs and priorities (Juma and Konde, 2013). The rise of off-patent, generic tools and the lowering of technical barriers for engineering biology has the potential to change this, particularly where new foundational advances are made open for others to build on (Hope, 2008). This is demonstrated in open source software and, more relevantly, in drug discovery (Masum, 2011). Current examples in biotechnology include the work of New Harvest, a US non-profit organisation that is building a library of open source cell lines for cultured meat production, and numerous open source providers of open hardware that enable high-throughput experimentation, such as the OpenTrons liquid handling robot and DropBot digital microfluidics system. Although platforms such as espacenet.com and lens.org help promote transparency, the patent landscape for engineering biology is complex (Carbonell et al., 2016; Carlson, 2011). Publicly available resources clarifying the status of open source biotechnologies could provide great benefit for enhancing the public’s return on investment in research and in the patent system itself. Leveraging open source biotechnologies – those that entered the public domain via the patent system as well as those made available through legal tools such as the BioBrick Public Agreement and OpenMTA – could facilitate widespread sharing of knowledge and foundational tools for engineering biology (Grewal, 2017).
Many governments see a thriving bioeconomy as the basis of national prosperity in the 21st century, and synthetic biology will be a key component of the infrastructure needed to deliver this (Carlson, 2016). The UK Synthetic Biology Leadership Council (SBLC) Strategic Plan (Synthetic Biology Leadership Council, 2016) focuses attention on the translation of emerging ideas and commercialisation of applications with the target of a £10 billion synthetic biology based platform in the UK by 2030, building on maximizing the capability of the innovation pipeline, building an expert workforce, developing a supportive business environment, and building value from national and international partnerships. A diverse, widely distributed and varied infrastructure will be critical, in the sense of being essential to the delivery of the expected benefits from the bioeconomy, and as such worthy of protection. However, the bioeconomy will subsume many sectors in a nation’s economy (pharmaceuticals and health care; energy and transport; agriculture, food and fibre production; water and waste management; and potentially electricity generation). Vulnerability to criminal or terrorist activity is a legitimate concern, but the widely dispersed nature of this infrastructure (geographically and sectorally) will put it in a different category from what are currently considered National Critical Infrastructures (Lewis et al., 2013). More damage could therefore be caused to the bioeconomy by well-meaning attempts to protect it from threats than by the threats themselves. Countries are concerned that any loss of competitive edge would seriously impact their national security, via economic opportunity costs and the impeded development of specific security applications, such as developing medical countermeasures to threats and improving diagnostics (Gronvall, 2015). Picking the appropriate governance modalities will require balancing the freedom to innovate against the security benefits of centralisation and control (International Risk Governance Council, 2011).